Like people, bacteria have their preferences when it comes to relationships. Some are totally independent, while others prefer company. Salmonella and many other kinds of bacteria are of the social type: They can live and even thrive inside a host cell. But unlike us, these bacteria do not spend a long time wooing the cell in the hope that it will welcome them in. Instead, they inject proteins that take control of the host cell’s systems.
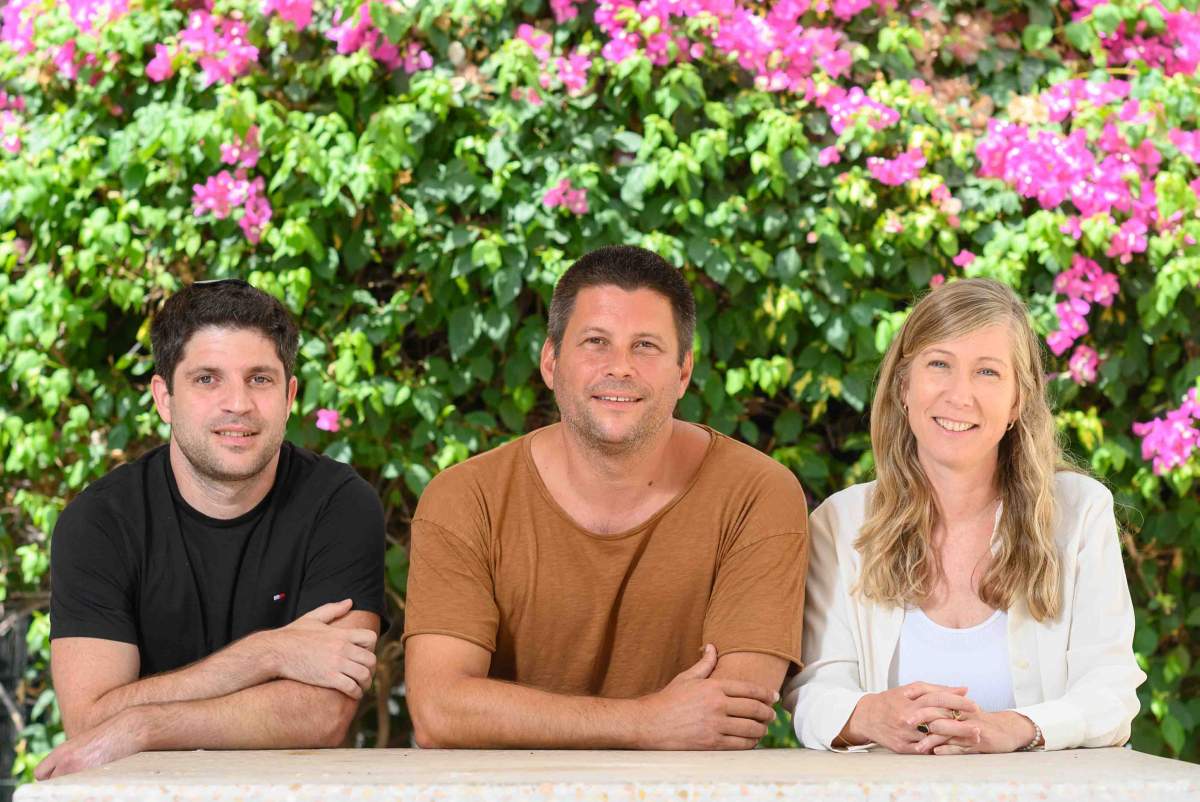
In recent years, thanks in part to studies conducted by Prof. Roi Avraham’s team at the Weizmann Institute of Science, researchers have identified differences among the proteins that various bacterial subspecies inject into their hosts, which could explain why some of these subspecies are more virulent than others. For example, there are more than 2,500 subspecies of salmonella, but only a handful of them cause life-threatening disease. In a paper published in the Proceedings of the National Academy of Sciences (PNAS), scientists from Avraham’s team presented a new research method that shines fresh light on the relationship between bacteria and their host cells – and reveals what makes some bacteria particularly virulent.
Over the past decade, scientists have gained the ability to examine molecular processes at an unprecedented resolution, revolutionizing research in the life sciences. New methods of sequencing genetic material on the single-cell level have contributed to this revolution. But existing tools cannot be used to apply single-cell RNA sequencing to examine all the molecular relationships between thousands of bacterial subspecies and the no-less impressive array of hosts. To map the differences in virulence and disease-causing ability between the various subspecies of salmonella, for example, researchers need to sequence the DNA of the bacterial cells on the single-cell level, do the same for the DNA of the specific host cells that were infected and match up the findings of guest and host, which amounts to a daunting task.
""We discovered how bacteria manipulate host cells and how cells respond, which may help treat antibiotic-resistant infections"
The new approach, developed in Avraham’s laboratory in Weizmann’s Immunology and Regenerative Biology Department, achieves just that, thanks to two key elements. The first, a method created by researchers led by Dr. Ori Heyman, consists of taking a group of bacterial species, each of which has a genetic mutation, and marking them with barcodes, DNA sequences that allow the scientists to identify each species later in the study, even when it is inside the host cell. The second element, a computer model called MAESTRO, developed by researchers led by Dr. Noa Bossel Ben-Moshe, pairs the sequencing results of each bacterial barcode with the sequencing results of the specific host cell that the bacteria have infected. Using this model, researchers can analyze exactly how each mutant bacterial species affects the behavior of the host and which proteins are expressed exclusively in those host cells that have been infected by a certain species of bacteria. This paves the way for the “paired sequencing” of the bacteria-and-host “couple” on the single-cell level.
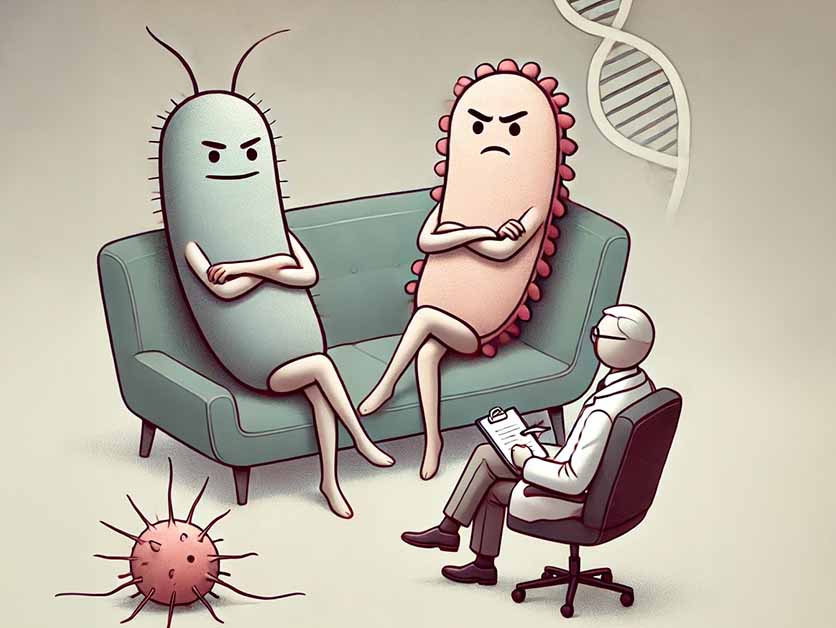
In their study, the researchers applied their new method to 25 mutant salmonella species that infected immune system cells called macrophages. The method allowed them to study the relationship between each species of bacteria and its host cell and to identify a species that causes an exceptionally powerful immune response in the host. The species in question lacks a protein that the other bacteria express and successfully inject into the host. The researchers inferred that this protein is essential for repressing the host’s immune system. “We discovered a new role of a familiar protein and showed that it sabotages the host’s defense mechanisms,” Avraham explains. “In fact, bacteria inject many proteins into their hosts, and we still have not figured out which roles most of them play. Our method, which is already used by researchers around the world, will make it possible to continue systematically revealing these roles. Moreover, it can be applied to any kind of bacteria, including friendly bacteria that are vital to many of our body’s systems.”
Beyond advancing basic research, the new method might help develop ways of battling bacterial resistance to antibiotics, defined by the World Health Organization as one of the main threats to human health and food security. “There are two new potential defense strategies, both still in the early stages of development,” Avraham says. “One is reducing the virulence of disease-causing bacteria and the other is bolstering the immune response of the host cells. Our method makes it possible to study both: simultaneously understanding how the bacterium launches its attack and how the host cell defends itself.”